The Light Gas pistol at the University of Kent is a cumbersome apparatus that appears more like a lathe to me than a pistol.
Despite its sluggish look, the cannon can fire projectiles at 1.5km per second, or roughly 3,500mph, which is nearly twice the speed of a bullet.
Today, it was loaded with a piece of basalt rock the size of a pea, which would be blasted at a highly particular gel.
The gel is made of the protein talin, or a variation of the protein that has been refined and altered to give it an exceptional ability to absorb impacts, as we will see.
We are herded out of the gun room, and after a brief countdown, the gun operator, Luke Alesbrook, presses the trigger, activating the gun.
As the target is removed, smoke wafts from the rifle barrel. The gel has been shifted around a little but, miraculously, is still intact.
It is critical that the metal plate behind the gel is not destroyed. The basalt would have ripped a chunk out of the plate if it hadn’t been for the gel.
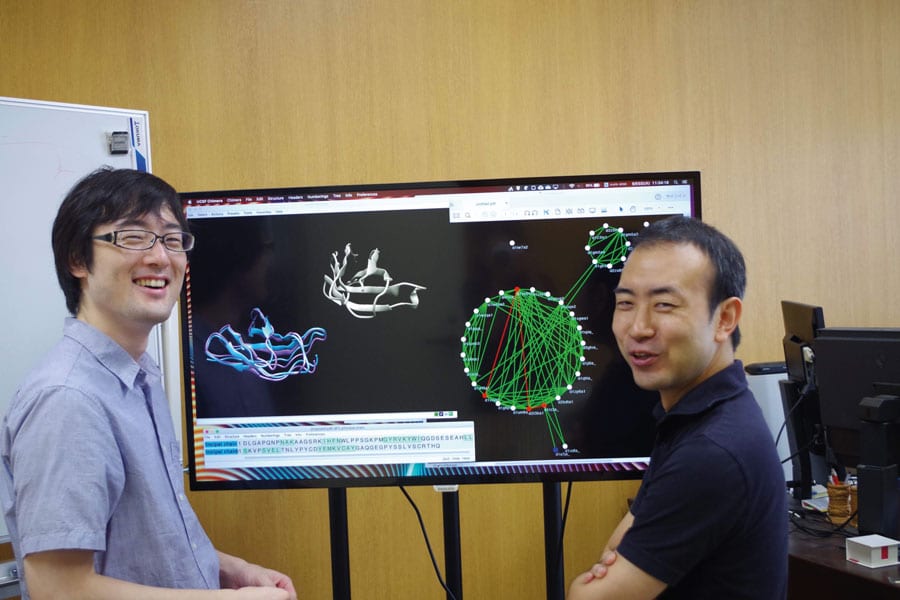
Because of its unusual mechanical qualities, talin can absorb force. Its structure consists of spirals of amino acids (protein building components) that form bundles. When pushed, the bundles unfurl, expanding the protein’s length by a factor of ten.
When the stress is released, the bundles return to their original position, similar to a spring.
Prof Ben Goult studied the structure of talin and how it responds to stresses, and he and his colleague Prof Jennifer Hiscock devised a method to turn talin into a shock-absorbing material.
“I just happened to walk into Ben’s office while he was talking about his fantastic protein.” “And I told them we had to make a bulletproof vest – that’s what we had to do,” she adds.
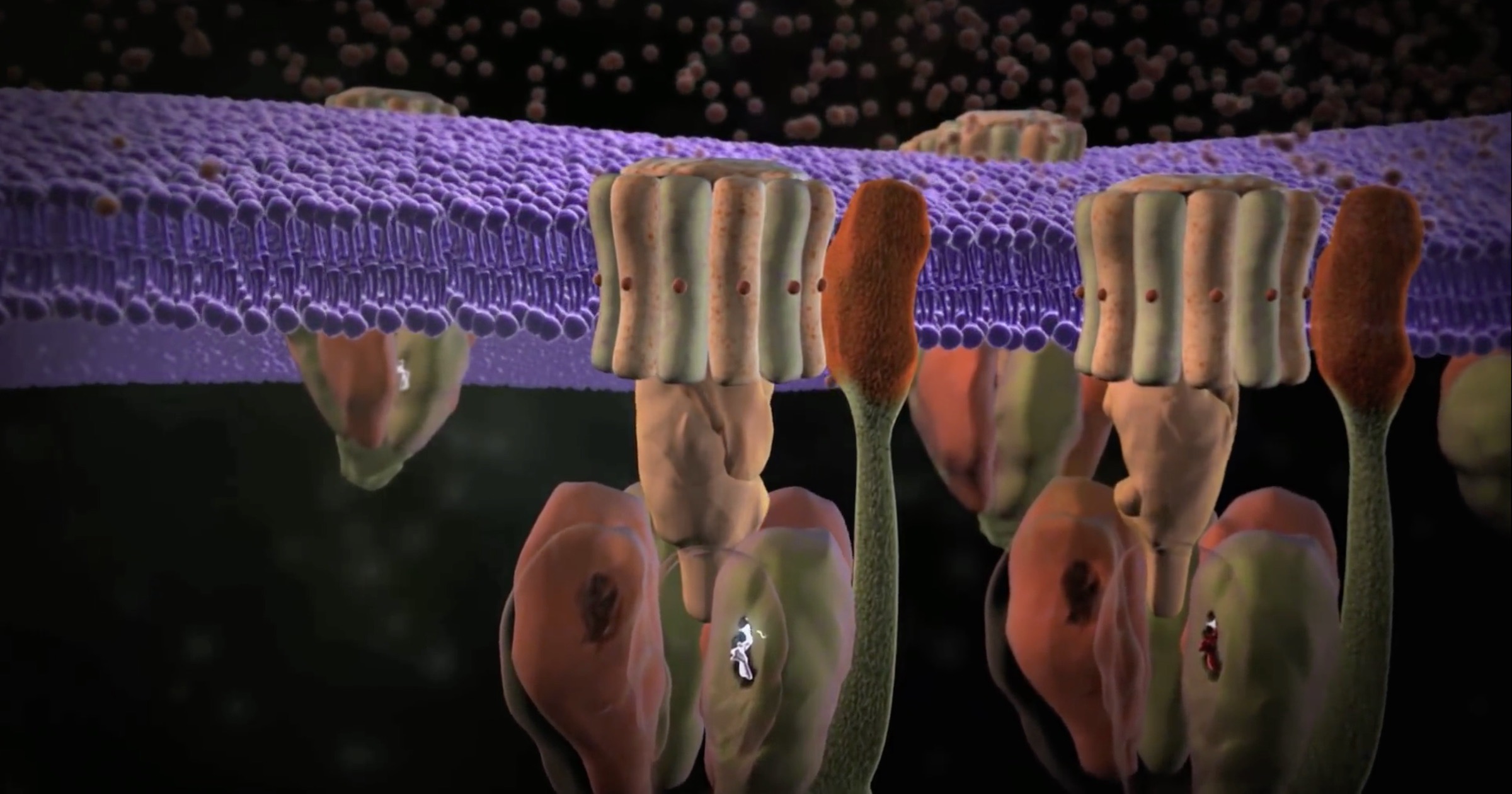
Beginning in 2016, their team discovered a mechanism to connect the talin proteins into a lattice, similar to a net with a cartoon-like ability to stretch and rebound.
Prof. Goult has been focusing on determining the mechanical properties of talin and its structure since 2005.
“It wasn’t simple. “It took four years for a team of six of us to figure out the protein structure of talin, and another four years to figure out how talin responded to force,” he explains.
Proteins are difficult to understand compounds. They are composed of a chain of amino acids, similar to beads on a string. Because there are 20 different naturally occurring amino acids – or beads – there are an infinite number of possible combinations.
Traditionally, such structures were determined using electron microscopy and X-ray crystallography, which may take years.
However, artificial intelligence (AI) has transformed the process in recent years, predicting the structures of hundreds of millions of proteins.
A significant event occurred in November 2020, when AlphaFold achieved best at CASP 14, a biannual assessment in which multiple computer programs estimate the structure of proteins.
Not only did AlphaFold outperform other algorithms, but it also predicted the structure of the proteins with far greater accuracy than its competitors.
“That was pretty crazy,” says Kathryn Tunyasuvunakool, who worked on AlphaFold with others at London-based DeepMind, Alphabet’s parent firm Google’s AI business.
“We knew we had relatively excellent internal performance going into the CASP. However, it was unclear whether other persons would experience comparable outcomes. “I guess it came as a surprise to us how large the margin was compared to other groups,” Ms Tunyasuvunakool said.
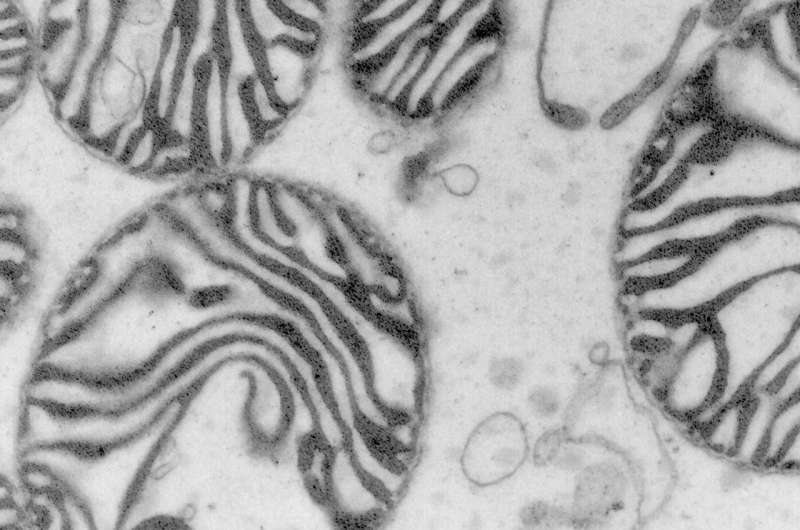
That version of AlphaFold, AlphaFold2, was so good that it was adopted by all of the top-performing teams in the following competition.
The protein structure database has grown from a few hundred thousand to hundreds of millions as a result of AlphaFold and its children.
This is a gold mine for scientists and researchers, particularly those involved in medication development. Proteins with structures that appear promising for particular applications, such as binding to a cancer cell, can be identified faster than ever before – the speed of research has accelerated.
However, even AlphaFold has restrictions. Proteins frequently interact with other molecules, yet AlphaFold can now only predict the protein part.
Proteins, as Prof Goult discovered with talin, are dynamic molecules that change shape. AlphaFold can provide researchers with a static picture but cannot model changes.
Scientists may also seek to create proteins from scratch to do specific tasks. Prof David Baker, director of the Institute for Protein Design at the University of Washington, just outside Seattle, is working on this.
His team created an artificial intelligence based on DALL-E, an AI that creates unique visuals.
RF Diffusion was trained by disassembling known proteins and then reassembling them in phases.
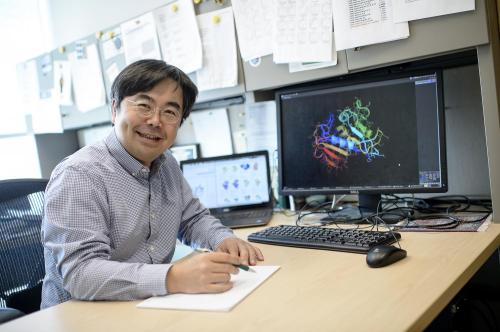
Scientists utilizing RF Diffusion decide on a specific feature they desire from a new protein, like as a catalyst for a specific type of chemical reaction or a protein that can attach to a specific target.
They can feed their specifications into RF Diffusion, and it will generate a suitable protein structure.
“I think RF diffusion is a step change compared to what we had before,” Prof Baker explains.
“The potential of designing proteins that solve so many different problems is really great,” he adds.
He anticipates the development of new medicines for cancer, neurodegeneration, and infectious disorders. Catalysts that can accelerate or improve the efficiency of reactions may be useful in industry.
Furthermore, according to Prof Baker, entirely new materials may develop. Protein study appeals to him because of the possibilities.
“It’s a very exciting area right now.” “It’s probably one of the most exciting areas in science today for young scientists just starting out,” he says.
Back in Kent, Professors Goult and Hiscock are collaborating with the Ministry of Defence to scale up manufacturing of their protein talin.
The plan is to produce enough shock-absorbing gel for a much larger test. The Kent researchers believe that their gel will one day be able to minimize the quantity of heavy ceramic utilized in today’s bulletproof plates.
Prof Hiscock is still amazed at how their protein gel comes together for the time being.
“It’s a beautiful process, the spontaneity of it, and the fact that all those molecules can come together and form those fibrous networks.”
Download The Radiant App To Start Watching!
Web: Watch Now
LGTV™: Download
ROKU™: Download
XBox™: Download
Samsung TV™: Download
Amazon Fire TV™: Download
Android TV™: Download